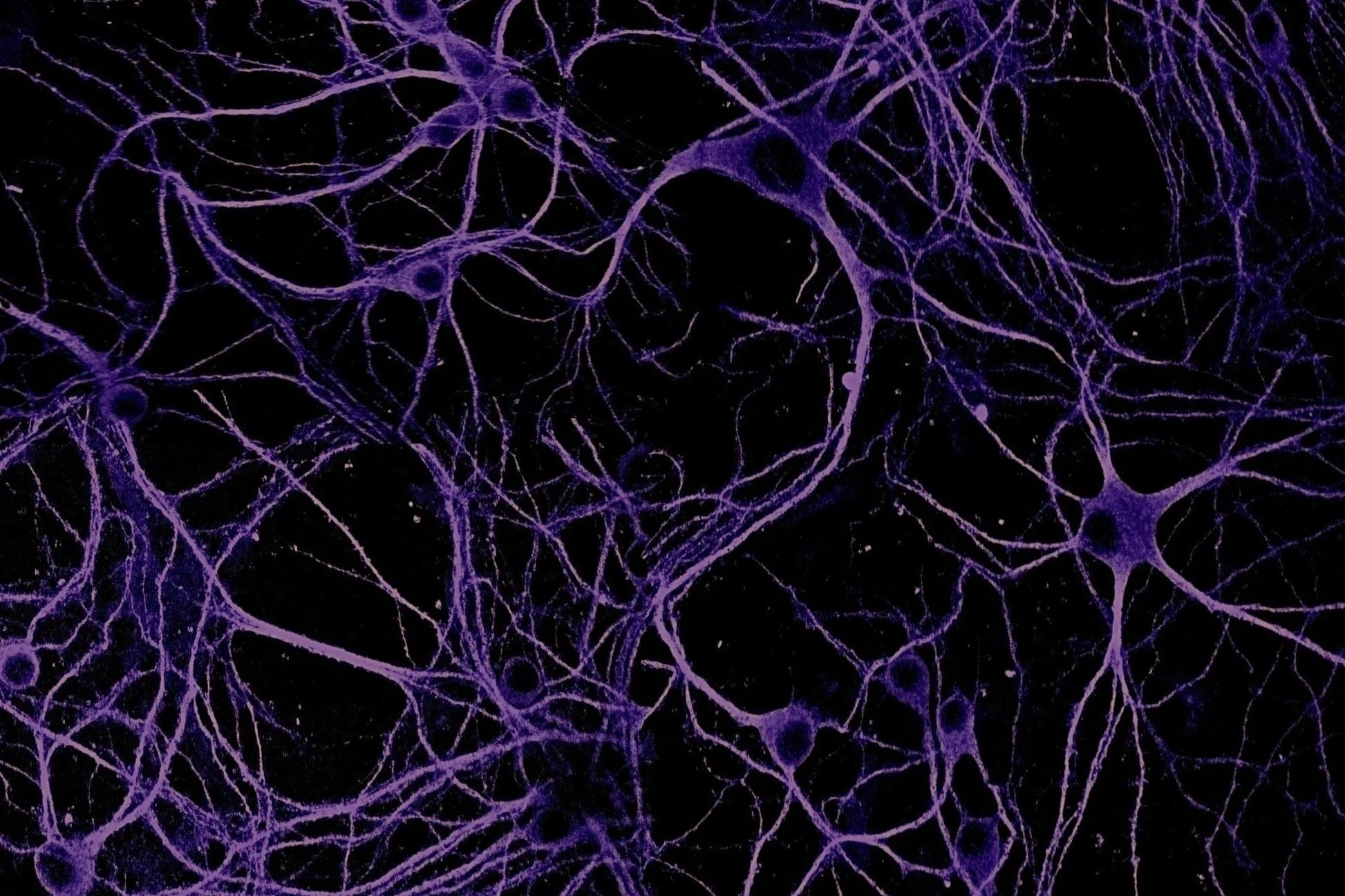
Research
We are curious about how neurons communicate. Neurons transfer information extremely rapidly (in milliseconds!) via contact sites called synapses. At the synapse, the presynaptic neuron (afferent) communicates to the postsynaptic neuron (efferent) via the release of neurotransmitters. Neurotransmitters are stored in small vesicles, called synaptic vesicles, in the presynaptic axon and are released via exocytosis. After release, neurotransmitters bind to specific ionotropic and metabotropic receptors at the postsynaptic membrane, usually in the dendrites of the postsynaptic neuron. Activation of the neurotransmitter receptors generates an electrical signal in the postsynaptic neuron that travels along that neuron and onto the synapses with the next neuron in the network, thus passing on information across the nervous system.
We use molecular and pharmacological tools combined with live fluorescence imaging, super-resolution microscopy and electrophysiology of cultured neurons to investigate molecular mechanisms that regulate neurotransmission at the level of single synapses. These mechanisms include synaptic vesicle fusion and endocytosis, calcium signaling, transport of receptors and other molecules, remodeling of synapse morphology, among others.
Calcium changes in the Endoplasmic Reticulum
Calcium homeostasis and calcium signals modulated by the stores
During neuron firing, the release of neurotransmitters, and thus normal functioning of synapses, is strictly dependent on calcium influx into the axon via voltage-gated calcium channels. These channels, however, are not essential for calcium signaling at rest and calcium homeostasis in neurons.
The major reserve of calcium in all cells is the endoplasmic reticulum (ER). The neuronal ER is particularly large, since it comprises a continuous organelle that spans the whole neuron volume (from cell body to distal dendrites and axon). Calcium uptake by the ER helps buffer excess calcium during neuron activity. Calcium release from the ER contributes to activate neuronal signaling pathways during different forms of neurotransmission and synaptic plasticity.
We are currently focused on a specific ER-related calcium pathway: store operated calcium entry (SOCE). This pathway is activated by changes in the calcium content inside the ER and it leads to influx of extracellular calcium into the cytoplasm. We have previously shown that SOCE modulates the release of the neurotransmitter glutamate and it contributes to calcium dyshomeostasis and neuron degeneration in chronic stress. But much remains to be understood. Some questions we are investigating are: When is SOCE engaged during neuronal activity? How does SOCE contribute to neurotransmission? Is SOCE activation beneficial or detrimental during neurodegeneration? To answer these questions we are combining different microscopy techniques, to gain a molecular understanding of the spatiotemporal dynamics of the calcium signals and the proteins involved, with electrophysiology.
Calcium changes in dendritic spines
Why do we care about Calcium?
Some examples…
Miniature excitatory postsynaptic currents (minis)
>>>>> What happened?? >>>>>
We triggered Calcium influx via SOCE and it caused an increase in minis!!
Spontaneous action potential firing by a pyramidal hippocampal neuron
>>>>> What happened?? >>>>>
We removed extracellular calcium, essential for action potential generation
Calcium is a key signaling molecule that modulates many forms of neurotransmission and its dysregulation leads to neurodegeneration
Neuron communication via extracellular vesicles
While neuron firing and synaptic transmission mediate extremely fast communication and information transfer among neurons, there are slower modes of communication that involve the secretion of signaling molecules and other small structures, and mediate homeostatic, functional and metabolic adaptations. One of the newest and least understood extracellular signals in neurons are extracellular vesicles. These are small membranous structures (similar to an intracellular organelle) that contain neuronal genetic materials, proteins and lipids. We have recently found that neuronal extracellular vesicles can enhance neurotransmitter release at synapses, via a pathway dependent on the SNARE synaptobrevin-2 (VAMP2) and the tetraspanin CD81. Other labs have also demonstrated that extracellular vesicles carry markers of neurodegeneration and are capable of propagating deleterious signals to healthy parts of the brain (triggering aggregation of proteins like Tau and Amyloid-beta, for example). These extracellular vesicles also have a great potential for targeted delivery of molecules to neurons. In order to fully understand all these processes, and hopefully leverage that knowledge for drug delivery, we first need to gain a molecular understanding of how they work. So our lab’s goal is to understand the molecular mechanism of formation, secretion, and incorporation of these neuronal extracellular vesicles and their effects on neurotransmission.